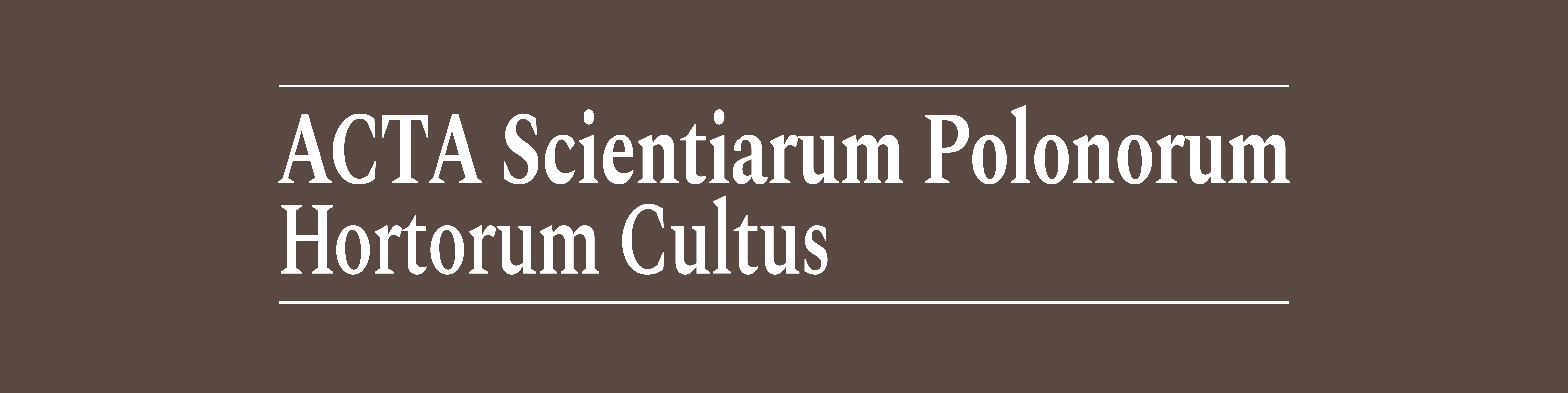
Vitex trifolia Linn. var. simplicifolia Cham is a medicinal aromatic plant and perennial halophyte growing in the coastal areas around the Bohai Sea in China. The aim of this study was to investigate the salt tolerance mechanisms of V. trifolia when subjected to different concentrations of NaCl (0, 90, 180, 270, 360 and 450 mM) by measuring growth parameters, ion contents, proline, soluble sugar, soluble protein, malondialdehyde (MDA), photosynthetic pigment contents, chlorophyll fluorescence parameters and antioxidant enzyme activities. The plants died when the NaCl concentration reached 450 mM 20 days after salt stress. Biomass and shoot growth were inhibited by increasing salinity, while root growth was promoted at a NaCl concentration ranging from 90 to 270 mM. Na+ and Cl– accumulation was markedly promoted in both leaves and roots with increasing salinity, while no significant changes were observed in the K+ concentration and K+/Na+ ratio in the leaves. Proline, soluble sugar and soluble protein contents increased significantly with increasing salinity. In order to eliminate the reactive oxygen species (ROS) produced by salt-induced oxidative stress, the activities of peroxidase (POD), catalase (CAT) and ascorbate peroxidase (APX) were enhanced. Photosynthetic pigment contents and PSII activity did not significantly decrease under salt stress. The results indicate that the mechanism of salt tolerance in V. trifolia are by ion homeostasis, osmotic balance, antioxidant enzyme induction and photosynthesis adjustment.
<< < 20 21 22 23 24 25 26 27 28 > >>
Możesz również Rozpocznij zaawansowane wyszukiwanie podobieństw dla tego artykułu.