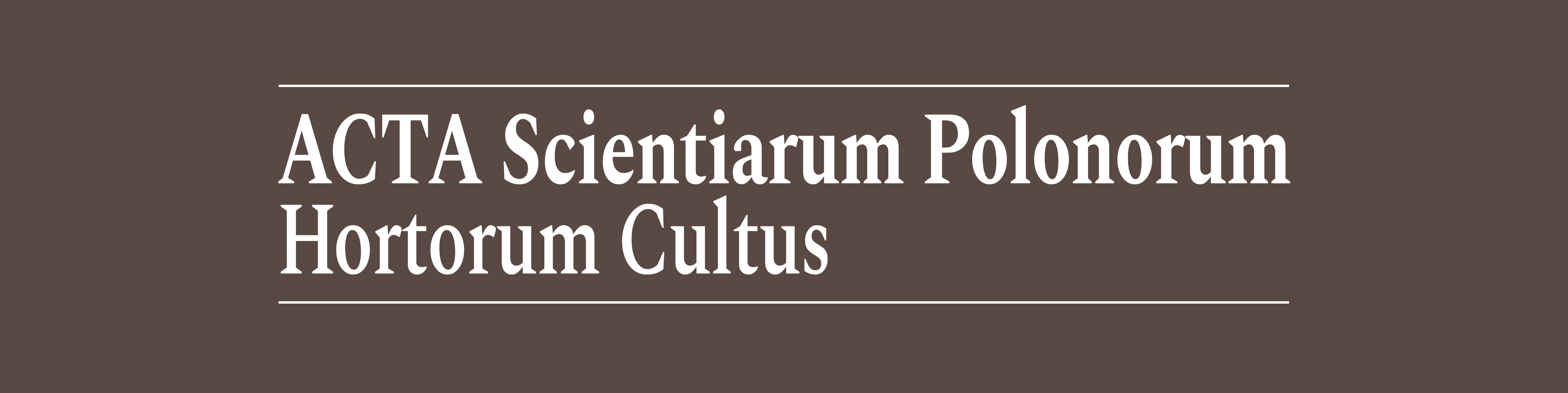
Bulbil germination is crucial to the survival of Chinese yam plants, the preservation of germplasm resources and the worldwide supply of food and natural medicine. There are still some unknowns regarding bulbil biochemical variations associated with germination. The metabolic changes during the germination of Chinese yam (Dioscorea polystachya Turczaninow) bulbils were studied using ultrahigh-performance liquid chromatography coupled with quadrupole time-of-flight mass spectrometry (UPLC-Q-TOF-MS) at eight-time points covering all four phases of germination. It was determined that 27 metabolites, including organic acids, amino acids, sugars, lipid metabolites, phenolics and steroids, were responsible for the variation in the Chinese yam bulbil groups. A metabolomics pathway was proposed based on the identified metabolites. The main processes affected during germination were those related to carbohydrate metabolism, the TCA cycle, lipid metabolism, nitrogen metabolism, lipid metabolism and polyphenol metabolism. It is one of the earliest reports on the metabolite identification and profiling of Chinese yam bulbils at different germination stages.
<< < 24 25 26 27 28 29 30 > >>
You may also start an advanced similarity search for this article.