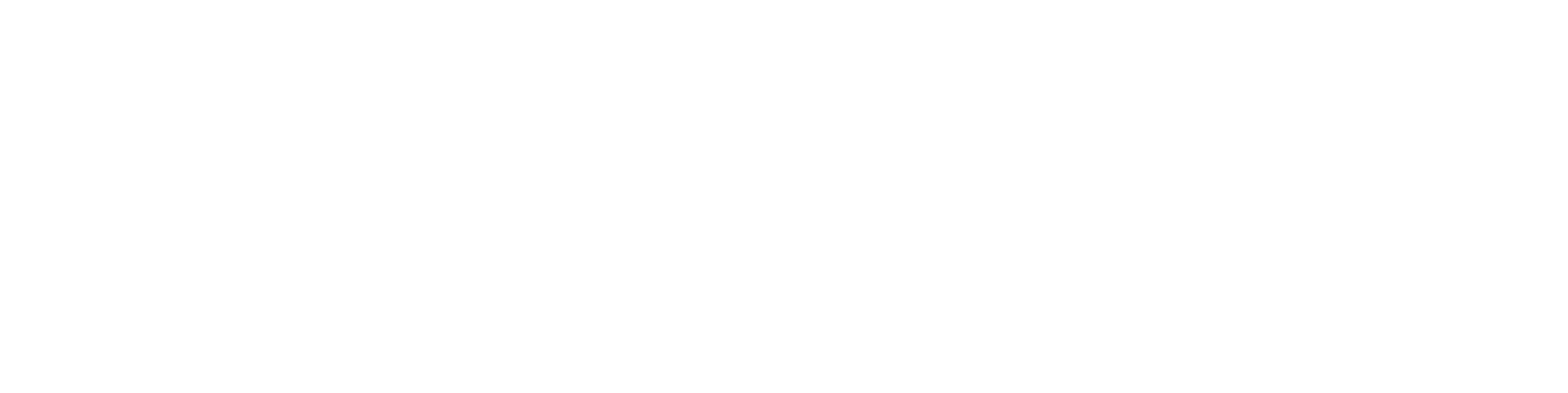
Agronomy Science, przyrodniczy lublin, czasopisma up, czasopisma uniwersytet przyrodniczy lublin
W ostatnich latach dokonał się ogromny postęp w zakresie poprawy odporności drzew leśnych na stres abiotyczny. Zastosowanie technik modyfikacji genetycznej pozwala na otrzymanie drzew odznaczających się dobrym wzrost w warunkach różnych czynników stresowych, takich jak wysokie zasolenie, susza, niska temperatura i zanieczyszczenie środowiska. Szczególnie podatna na modyfikacje genetyczne jest topola. Wobec nadchodzących zmian środowiskowych zastosowanie transformacji genetycznej w hodowli leśnej wydaje się być atrakcyjną perspektywą. W niniejszej pracy omawiamy najnowsze osiągnięcia dotyczące genetycznie modyfikowanych drzew charakteryzujących się zwiększoną odpornością na niesprzyjające warunki środowiska.
Możesz również Rozpocznij zaawansowane wyszukiwanie podobieństw dla tego artykułu.