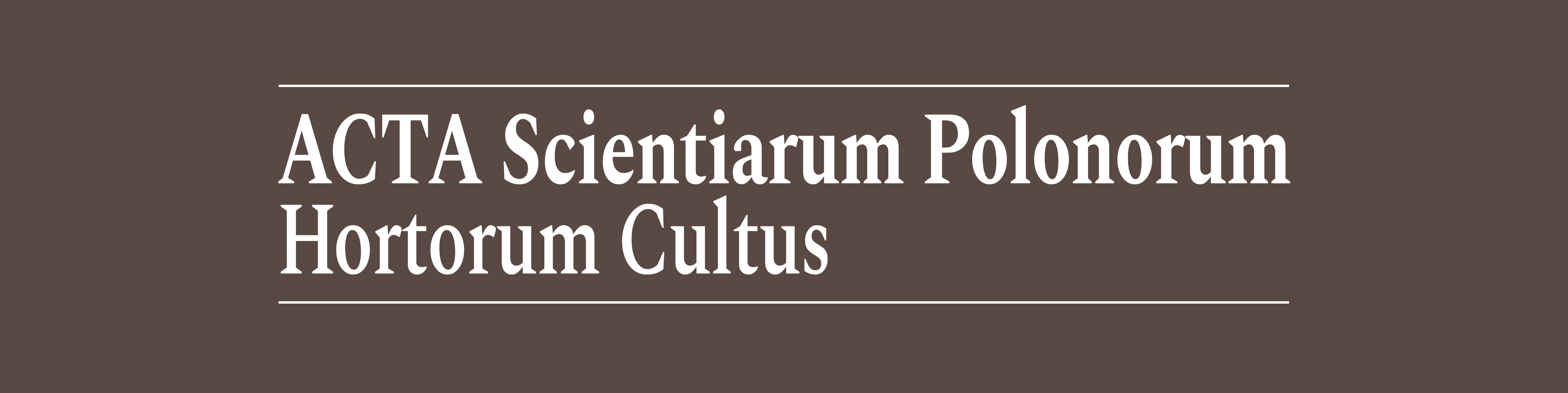
Karrikins are a group of chemicals found in plant-derived smoke from burning plant material. Till now, scientists concentrated on their effect on seed germination in plants sensitive to smoke. However, there are reports on the effect of karrikins on physiology and morphology in plants which do not occur in fire areas and are not naturally treated by smoke. These chemicals positively affect the biometric parameters of the in vitro cultured plants. Recently, their effect on germination rate of pollen in several species has been confirmed. They interact with plant growth regulators enhancing their effects, for example using them together with auxins, cytokinins, gibberellins, abscisic acid or ethylene. This paper contains a review of present research on karrikins and proposes perspectives of further investigations, as well as application of these chemicals in horticultural production as a new group of plant growth regulators.
<< < 12 13 14 15 16 17 18 19 20 21 > >>
You may also start an advanced similarity search for this article.